
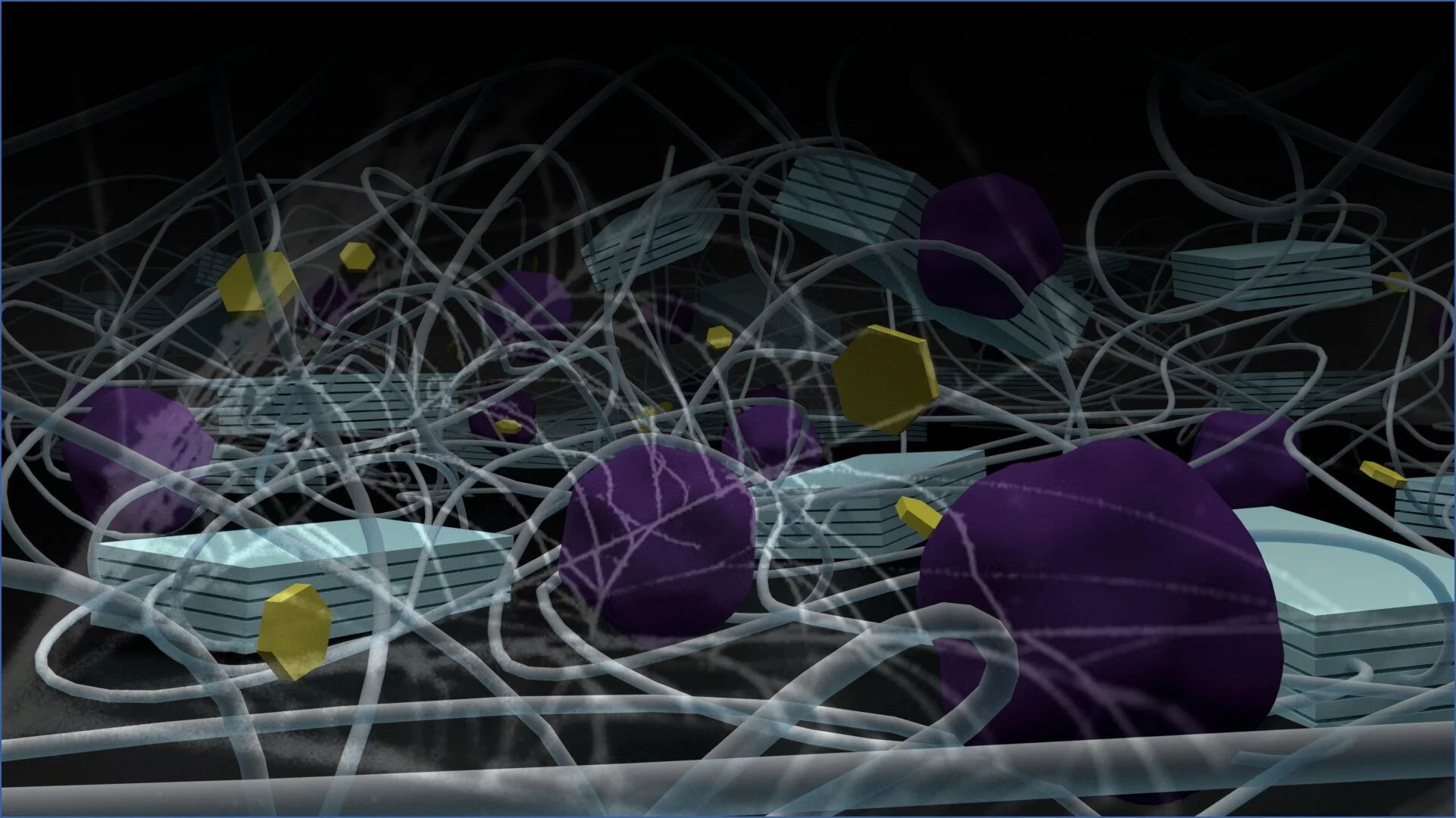
What we do
create new “bioinspired” and “Biomimetic” materials
The natural world is brimming with materials with extraordinary and complex properties, such as stimuli-responsiveness, high mechanical toughness, and the ability to function in extreme environments. Our research aims to create synthetic materials using molecular building blocks inspired by or derived from nature in order to mimic the desirable attributes found in natural materials. We utilize a wide range of covalent and non-covalent chemistries to build these materials with molecular level precision. We also utilize a panel of advanced characterization tools to examine the structures formed by these biomimetic molecules from nano to macroscopic length scales.
innovate for human healthcare and environmental sustainability
As the population of our planet grows, the sustainability of our material consumption and the availability of affordable, effective healthcare technologies become paramount challenges facing society. Our research works to address these challenges by developing new innovations in materials synthesis and design. These innovations can lead to improvements in regenerative medicine and drug delivery, decrease the cost of drug development, and reduce recalcitrant plastic waste in the environment. Our work in these areas utilize a variety of approaches, ranging from bioprocess and metabolic engineering to chemical synthesis to computational simulation. Where possible, we seek to establish industry partnerships to maintain a pathway towards commercial relevance.
uncover the fundamental rules that govern natural materials
Many materials in nature are able to exhibit amazing, often paradoxical properties, in large part due to their chemical structure and composition. Importantly, the molecular level features of these materials “program” the assembly of structures, from nano to macroscopic length scales, resulting in functions not commonly observed in conventional man-made materials. Understanding how such assembly phenomena occurs enables us to rationally design next-generation materials with targeted properties. To that end, we study the inter- and intra-molecular interactions of naturally derived and biomimetic macromolecules in controlled environments to reveal fundamental rules governing their behavior. We leverage our findings towards developing new technologies to meet the materials needs of our advancing society.
Research Interests
self-assembled coatings that improve biomedical device performance
Non-specific adhesion of proteins to surfaces, also known as “fouling”, has long been considered a ubiquitous phenomenon that can lead to problems such as failure of devices that operate in aqueous environments, undesirable immune responses towards therapeutic interventions, and establishment of environments that promote the growth of drug-resistant microbes. However, our work leverages the persistence of protein fouling as a bottom-up approach towards increasing the function of a diverse range of materials. By controlling the balance between protein-surface adhesion and protein-protein assembly, we have developed a non-covalent method for generating adherent, defect-free nanothin coatings. These coatings can completely transform the physicochemical properties of a surface without harsh chemicals or complex instrumentation. We have demonstrated that our coating approach is particularly well-suited for improving the therapeutic efficacy of biomedical devices that have complex geometries, such as nanostructured scaffolds for tissue engineering. Our coating approach is also advantageous for materials that lack chemically reactive functional groups and for systems where the presence of biological components prohibits the use of standard covalent chemistries. Our current work aims to understand the fundamental mechanisms controlling coating formation and to expand the portfolio of functions enabled by our coating process.
Recent Publications:
Caleb Wigham, Tanner D. Fink, Mirco Sorci, Padraic O’Reilly, Sung Park, Jeongae Kim, Vrushali R. Varude, R. Helen Zha*. Phosphate-Driven Interfacial Self-Assembly of Silk Fibroin for Continuous Noncovalent Growth of Nanothin Defect-Free Coatings. ACS Applied Materials and Interfaces. 2024 (in press).
Tanner D. Fink, Jessica L. Funnell, Ryan J. Gilbert, R. Helen Zha*. One-Pot Assembly of Drug-Eluting Silk Coatings with Applications for Nerve Regeneration. ACS Biomaterials Science and Engineering. 2024, 10, 482-496.
Alexis Ziemba, Tanner D. Fink, Mary Clare Crochiere, Devan Puhl, Samichya Sapkota, Ryan J. Gilbert*, R. Helen Zha*. Coating Topologically Complex Electrospun Fibers with Nano-Thin Silk Fibroin Enhances Neurite Outgrowth In Vitro. ACS Biomaterials Science & Engineering. 2020 6 (3), 1321-1332.
R. Helen Zha*, Peyman Delparastan, Tanner D. Fink, Joschka Bauer, Thomas Scheibel, and Phillip B. Messersmith. Universal Nanothin Silk Coatings via Controlled Spidroin Self-Assembly. Biomaterials Science 2019 7 (2), 683-695.
engineered microbes for a sustainable plastics economy
The overwhelming majority of plastics used today are derived from petrochemical feedstocks. Even with the ideal application of conventional recycling strategies, these plastics can only be recycled a limited number of times before end-of-life disposal by incineration or dumping in landfills, leading ultimately to greenhouse gas emissions, pollution and disruption of natural ecosystems, and loss of feedstock material. For the sustainability of mankind’s endeavors, future manufacturing will need to focus on materials with closed-loop lifecycles rather than the traditional linear extract-process-consume-dispose paradigm. Our research aims to develop new technologies and conceptual frameworks that enable a regenerative circular economy for the plastics industry that utilizes protein-based polymers. By leveraging the diversity of amino acid building blocks and the synthetic precision afforded by genetic templates, these polymers can potentially exhibit tunable properties targeted for a broad range of applications. The goals of our work are multi-faceted. We are engineering new microorganisms that can upcycle recalcitrant plastic into high-value protein-based bioplastics. We are also using computational and experimental methods to understand the relationship between protein sequence and material structure and properties across hierarchical length scales. Ultimately, our research works to transform the non-degradable plastic waste generated by our current economy into robust biobased materials for use in the renewable, waste-free economy of the future.
Watch a recent RED Talk presented by Dr. Zha on this research topic: https://www.youtube.com/watch?v=KbRBvnSLxY0&t=696s
Recent Publications:
Alexander J. Connor, Mattheos Koffas, R. Helen Zha*. Production and Secretion of Recombinant Spider Silk in Bacillus Megaterium. Microbial Cell Factories. 2024, 23, 35.
Alexander J. Connor, Jessica Lamb, Massimiliano Delferro, Mattheos Koffas*, R. Helen Zha*. Two-Step Conversion of Polyethylene into Recombinant Proteins Using a Microbial Platform. Microbial Cell Factories. 2023, 22, 214.
Alexander J. Connor, Caleb Wigham, Ying Bai, Manish Rai, Sebastien Nassif, Mattheos Koffas, R Helen Zha*. Novel Insights into Construct Toxicity, Strain Optimization, and Primary Sequence Design for Producing Recombinant Silk Fibroin and Elastin-Like Peptide in E. coli. Metabolic Engineering Communications. 2023, 16, e00219.
Zhengyang Xiao, Alexander J. Connor, Alyssa M. Worland, Yingjie Tang, R. Helen Zha, Mattheos Koffas*. Silk Fibroin Production in Escherichia coli is LImited by Positive Feedback Loop Between Metabolic Burden and Toxicity Stress. Metabolic Engineering. 2023, 77, 231-241.
silk-mimetic polymers with targeted materials properties
Silk has been a source of marvel for centuries as one of nature's high‐performance materials. Pound-for-pound, silk can be stronger than steel and tougher than kevlar. Furthermore, silk is a highly biocompatible material that is well-suited for biomedical applications, such as tissue engineering and drug delivery. The remarkable properties of silk primarily result from silk fibroin, a structural protein found in the core of silk fibers that can undergo supramolecular self-assembly. Our research aims to create synthetic polymers that mimic the chemical architecture and self-assembly behavior of natural silk fibroin. Such silk-mimetic polymers are of great emerging interest, as they can potentially exhibit the advantageous features of natural silk while possessing synthetic flexibility and scalability. To synthesize these silk-mimetic polymers, we utilize engineered microorganisms to create well-defined recombinant silk fibroin-like proteins. We are also exploring abiotic approaches, which utilize tools from polymer, organic, and peptide synthesis, to generate silk-mimetic polymers with additional functions provided by non-natural chemistries. We combine our synthetic work with computational simulation and multi-scale materials characterization, such as nano-scale microscopy and light scattering, in order to create materials with predictable properties. This project seeks not only to produce technologies enabling scalable production of silk-mimetic materials, but also to gain fundamental knowledge regarding the performance gap between silk and man-made polymers.
Recent Publications:
Amrita Sarkar, Cody Edson, Ding Tian, Tanner D. Fink, Katherine Cianciotti, Richard A. Gross, Chulsung Bae, and R. Helen Zha*. Rapid Synthesis of Silk-Like Polymers Facilitated by Microwave Irradiation and Click Chemistry. Biomacromolecules. 2021 22, 95-105.
Amrita Sarkar, Alexander J. Connor, Mattheos Koffas, R. Helen Zha*. Chemical Synthesis of Silk-Mimetic Polymers. Materials. 2019 12 (24), 4086.
Tanner D. Fink and R. Helen Zha*. Silk and Silk-Like Supramolecular Materials. Macromolecular Rapid Communications. 2018 39 (17), 1700834
Materials innovations that reduce viral exposure
Preventing and mitigating the effects of viral infections are important goals for modern healthcare. Because viruses are often less than 100 nm in size, eliminating viral exposure is especially challenging and requires unique materials innovations. Our research aims to develop technologies that filter and deactivate viruses, as well as potentially detect the presence of virus-like particles. Such technologies include antiviral polymer coatings that can be applied to filtration media, thus enhancing the performance and operating lifetime of personal protective equipment such as face masks. Other technologies include viral surrogate particles that can be used to rapidly assess the performance of critical processes used to remove viral contaminants in biopharmaceutical manufacturing. Because preventing viral infections is a global challenge faced by communities with disparate resources, our work in these areas emphasize ease-of-implementation as a design criteria. Thus, we utilize chemistries that involve non-toxic, commonplace reagents and processes which can be carried out using basic equipment. Our end goal is to create innovations that reduce viral exposure using simple strategies that respect our natural environment and require minimal re-tooling of existing manufacturing workflows.
Recent Publications:
Mirco Sorci, Tanner D. Fink, Vaishali Sharma, Sneha Singh, Ruiwen Chen, Brigitte L. Arduini, Katharine Dovidenko, Caryn L. Heldt, Edmund F. Palermo*, R. Helen Zha*. Virucidal N95 Respirator Face Masks via Ultrathin Surface-Grafted Quaternary Ammonium Polymer Coating. ACS Applied Materials and Interfaces. 2022, 14, 25135-25146.
Alexander J. Connor, R. Helen Zha, Mattheos Koffas*. Bioproduction of Biomacromolecules for Antiviral Applications. Current Opinion in Biotechnology. 2021 69, 263-272.
Funding
Our research efforts are graciously funded by private and public agencies.
Rensselaer Polytechnic Institute
National Science Foundation
NY Empire State Development Fund
Terapore Technologies, Inc
NIH National Institute of general medical sciences
the kavli foundation
New York Farm Viability Institute
Research corporation for science advancement

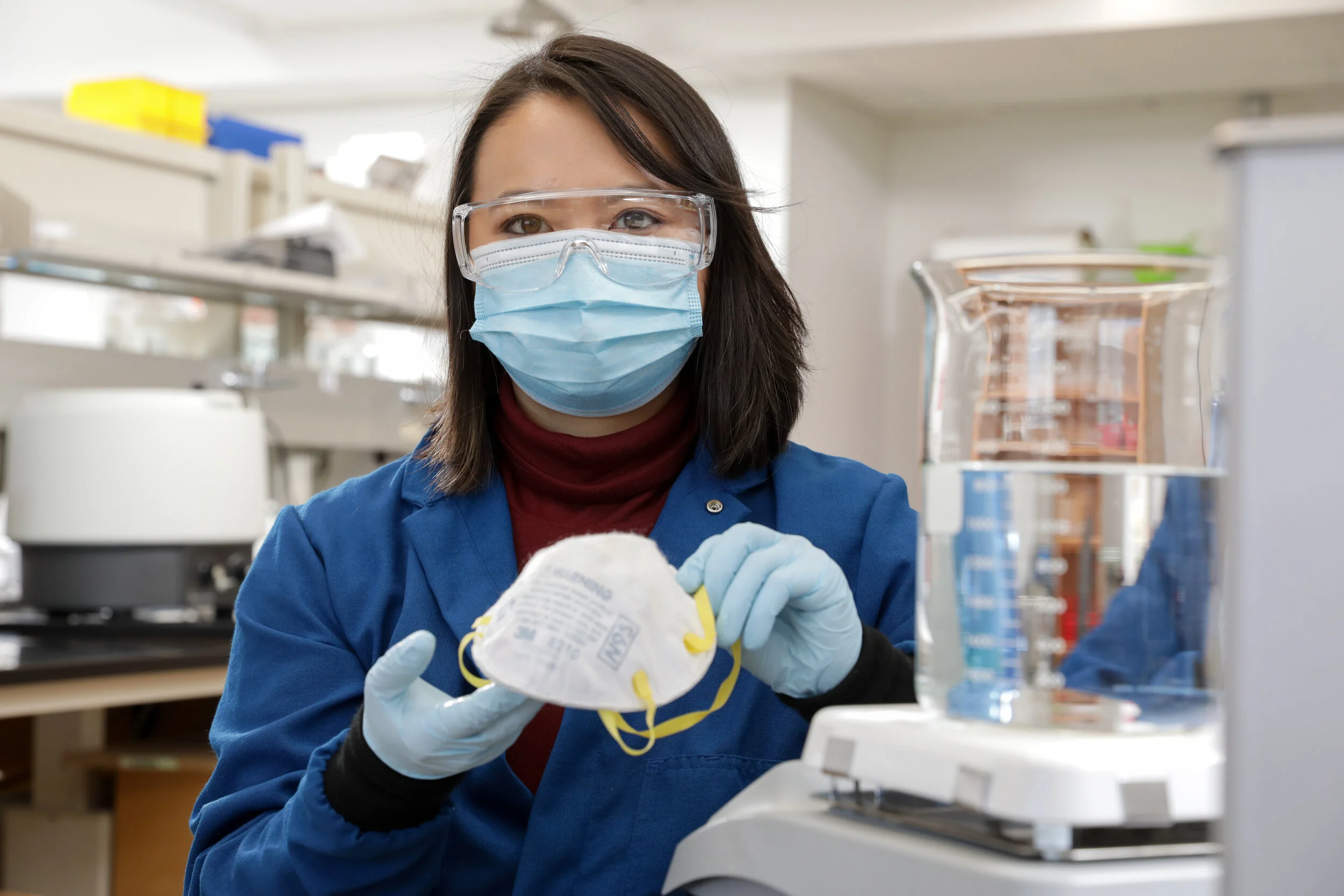
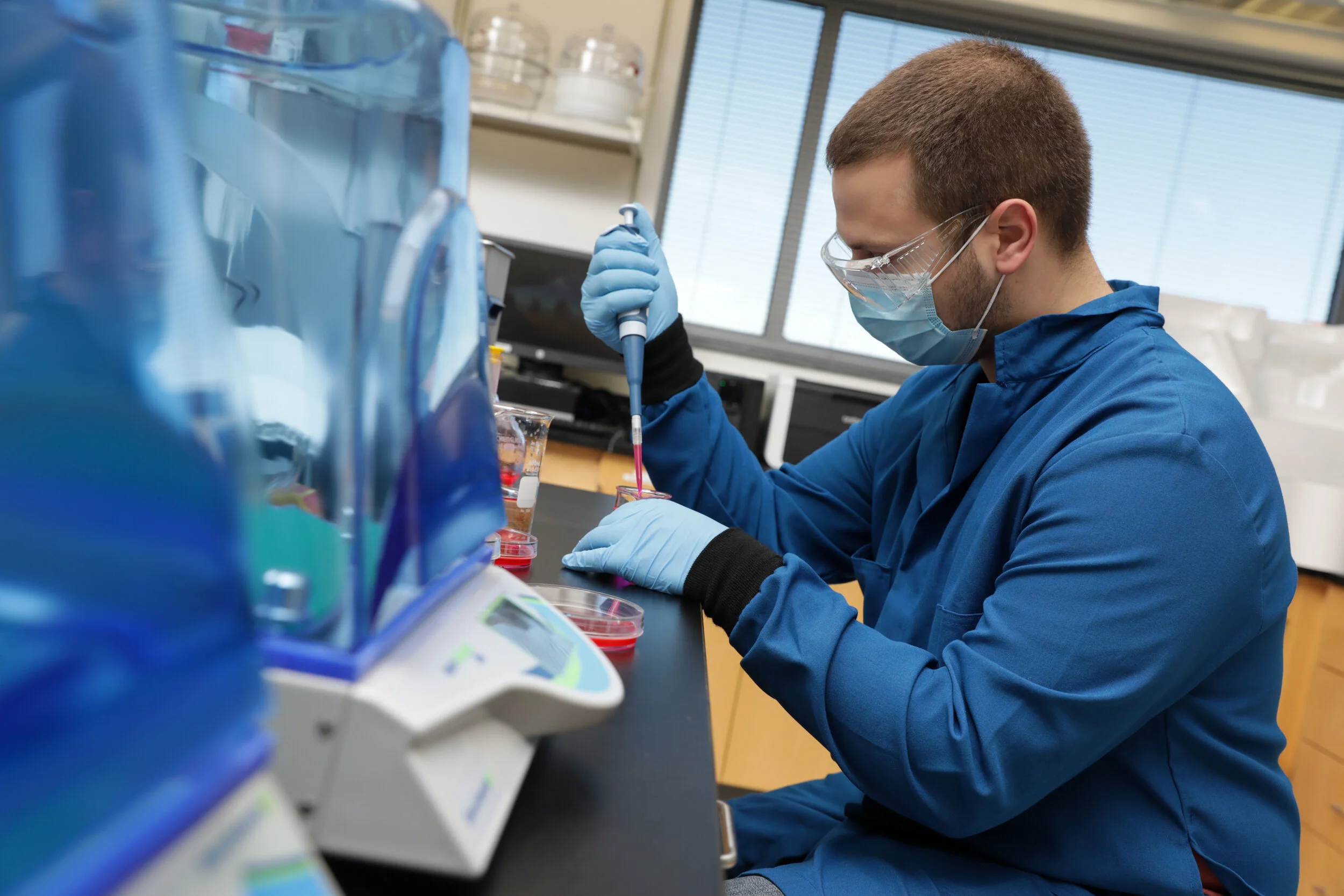
Want to get involved?
Contact Us
Stay Connected
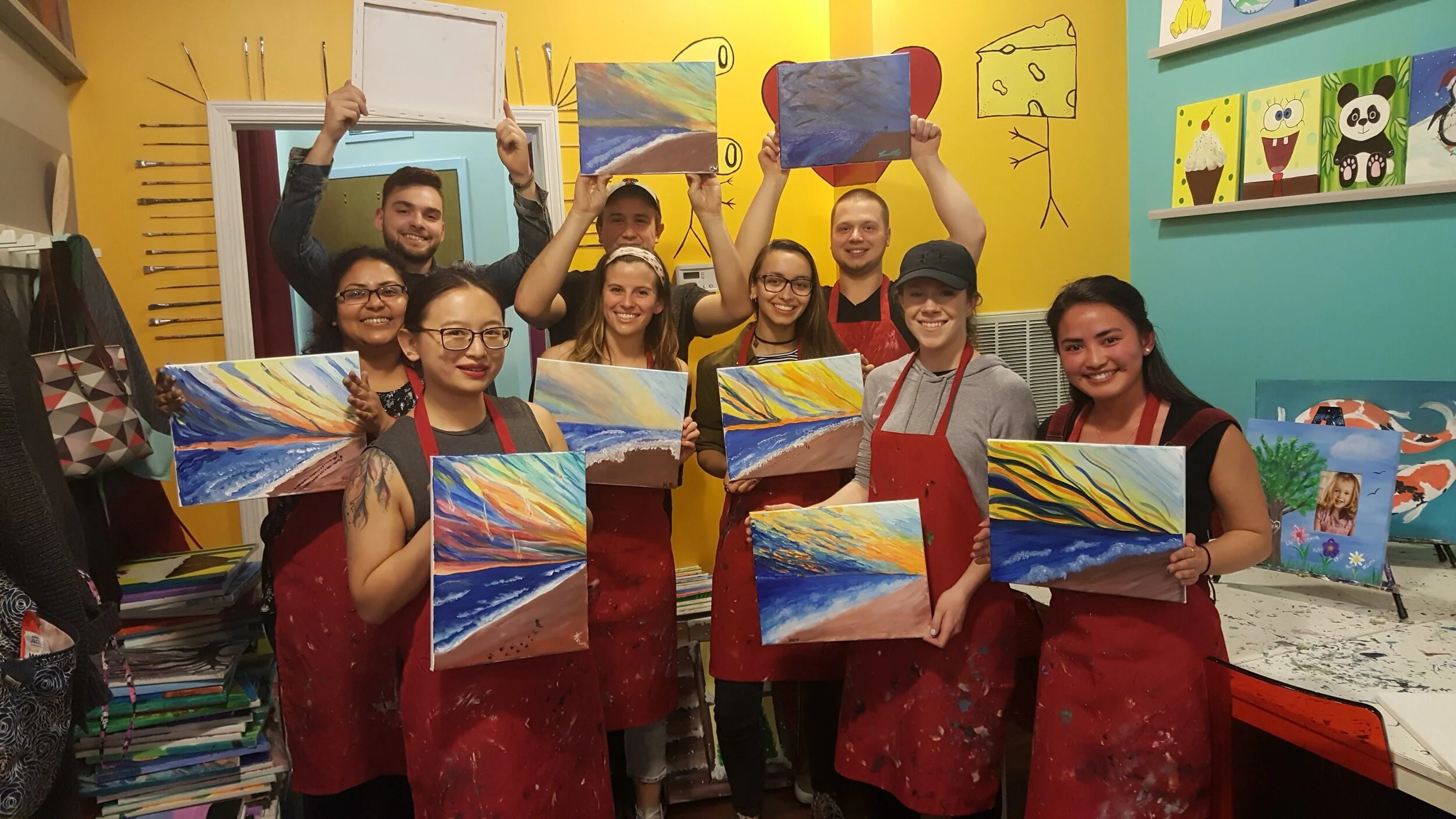